Our main purpose on the R/V Atlantis this summer will be to recover cores of deep-sea sediment in the North Atlantic, with the goal of reconstructing sea surface temperature over the past 10,000 years. But how is past sea surface temperature determined from a core of mud?
What’s an Alkenone?
The compounds of interest are alkenones. As the name suggests, these molecules are ketones that contain double bonds (alkenes) within their side chains. The ketone functional group consists of a carbonyl (C=O) attached to two hydrocarbon substituents (labeled R and R’) on the below diagram. In an alkenone, the R substituent is either a methyl or ethyl group, while the R’ substituent is a long (34-40 carbon) side chain, consisting of 2-4 double bonds in the trans (E) configuration (1).
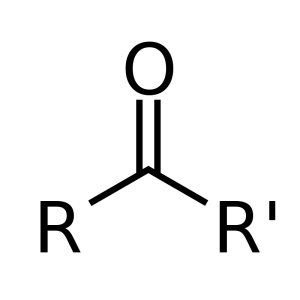

Alkenone Production
Alkenones were first identified in marine sediment in the late 1970s, and shortly afterward, it was determined that they are produced by specific species of haptophyte algae (2,3). The haptophytes are a small group of planktonic algae that obtain their energy from the sun (4). They are present across the globe in marine environments and represent significant primary producers (30-50% of total chlorophyll a biomass in oceans) (5). Alkenones are mostly produced by two specific species – Emiliana huxleyi and Gephyrocapsa oceanica (1).
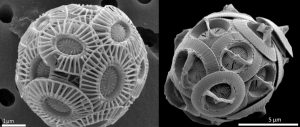
Alkenone Paleothermometry
So what’s the big deal with these compounds? In 1986, a research group out of the University of Bristol (UK) noticed that the distribution of alkenones in sea surface sediment differed based on latitude (6). It was eventually determined that alkenone-producing species respond to changes in water temperature by varying the types of alkenones they produce. Specifically, it was determined that at lower temperatures, E huxleyi and G. oceanica produce alkenones that are more unsaturated (contain more double bonds in their side chains). Scientists could now use the distribution of alkenones present in marine sediment to reconstruct sea surface temperatures of the past!
How is this done? Here’s a high-level overview. First, cores are extracted of deep-sea sediment and the different layers in the sediment are assigned ages. Alkenones are then extracted from layers of interest. Typically, sonnication-based solid-liquid extraction is used with a 9:1 methanol / methylene chloride liquid phase (1). Alkenone extract is then injected into a gas chromatograph. Within this device, the alkenones flow through a long column contained within an oven to bring them to boiling. Smaller, more volatile (lower boiling point) alkenones move faster through the column, while larger alkenones are more sluggish. Accordingly, the different types of alkenones are separated within the column. A flame-ionization detector records when each compound group leaves the column, and this data is recorded on a chromatogram plot.
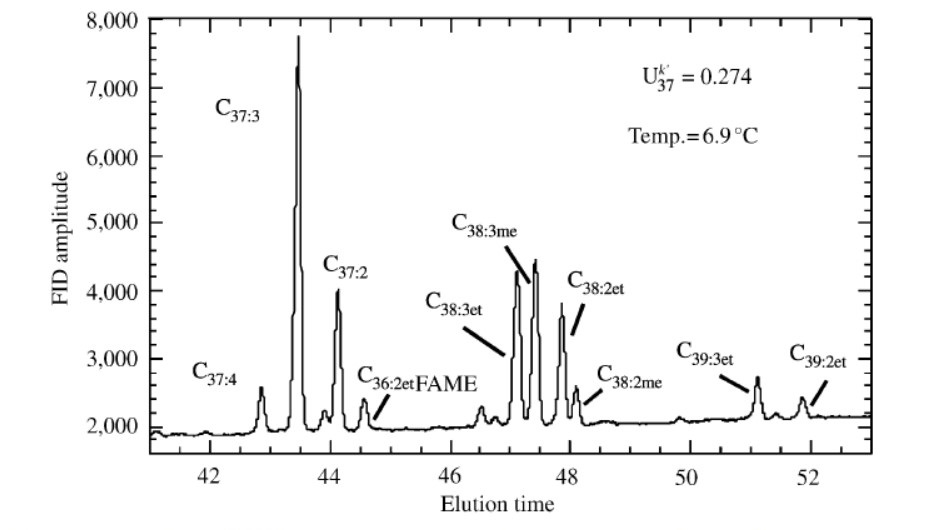
The X-axis on the chromatogram represents retention/elution time – the amount of time the compound spends in the chromatography column. The Y-axis represents the amount of each alkenone present in the sample – integration (area) of each signal correlates with its prevalence in the sediment.
A common way of reporting this data is called the U37Ko index, and it is determined from results for two specific alkenones – C37:2 and C37:3 (1). The 37 indicates that these are alkenones containing 37 total carbons. C37:2 contains two degrees of unsaturation (double bonds), which C37:3 contains 3. U37Ko is defined as C37:2 / (C37:2 + C37:3). This index varies positively with sea surface temperature – the higher the sea surface temperature, the greater proportion of C37:2 produced. Because the amount of C37:2 is normalized by C37:2 + C37:3, the index depends only on the relative proportions of these alkenones in the sediment, not absolute numbers. After determining U37Ko for a core sample from the geologic past, scientists can use calibration curve (based on data from sea surface samples around the globe) to estimate the temperature of the sea when the alkenones in the sample were produced.
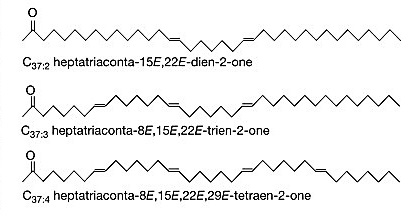
Did You Know?
While alkenones have been so useful to geoscientists, their function in E huxleyi and G. oceanica is not entirely clear. Some have suggested that they play a role in modulating cell membrane fluidity at different temperatures – similar to the role of membrane lipids in plants (7). This would account for changes in alkenone production based on sea surface temperature. However, alkenones haven’t yet been specifically associated with the membranes of E huxleyi and G. oceanica. Others have speculated that alkenones serve as energy storage molecules for the producing organisms (8). This makes sense, given that their structure resembles the fatty acid chain of a triglyceride. If they did serve simply as molecules for energy storage, though, why would production vary based on ocean temperature. The mystery remains!
Sources
- Herbert, T.D. “Alkenone Paleotemperature Determinations.” Treatise on Geochemistry, 2014, pp. 399–433, 10.1016/b978-0-08-095975-7.00615-x.
- Boon, J.J., et al. “Organic Geochemical Analyses of Core Samples from Site 362, Walvis Ridge, DSDP Leg 40.” Initial Reports of the Deep Sea Drilling Project, 38/39/40/41 Supl., Sept. 1978, 10.2973/dsdp.proc.38394041s.301.1978.
- Volkman, J.K., et al. “Novel Unsaturated Straight-Chain C37C39 Methyl and Ethyl Ketones in Marine Sediments and a Coccolithophore Emiliania Huxleyi.” Physics and Chemistry of the Earth, vol. 12, Jan. 1980, pp. 219–227, 10.1016/0079-1946(79)90106-x.
- Eikrem, Wenche, et al. “Haptophyta.” Handbook of the Protists, 2017, pp. 893–953, link.springer.com/referenceworkentry/10.1007%2F978-3-319-28149-0_38, 10.1007/978-3-319-28149-0_38.
- Liu, Hui, et al. “Extreme Diversity in Noncalcifying Haptophytes Explains a Major Pigment Paradox in Open Oceans.” Proceedings of the National Academy of Sciences, vol. 106, no. 31, 4 Aug. 2009, pp. 12803–12808, 10.1073/pnas.0905841106.
- Brassell, S. C., et al. “Molecular Stratigraphy: A New Tool for Climatic Assessment.” Nature, vol. 320, no. 6058, 1 Mar. 1986, pp. 129–133, www.nature.com/articles/320129a0, 10.1038/320129a0.
- Marlowe, I.T., et al. “Long Chain (N-C37–C39) Alkenones in the Prymnesiophyceae. Distribution of Alkenones and Other Lipids and Their Taxonomic Significance.” British Phycological Journal, vol. 19, no. 3, Sept. 1984, pp. 203–216, 10.1080/00071618400650221.
- Epstein, B.L., et al. “The Possible Metabolic Role of C37 Alkenones in Emiliania Huxleyi.” Organic Geochemistry, vol. 32, no. 6, June 2001, pp. 867–875, 10.1016/s0146-6380(01)00026-2.